All vaccines have the same basic goal: train the body’s defenses to recognize and respond to a specific threat, whether chickenpox, polio, or SARS-CoV-2 (the virus responsible for COVID-19).
To accomplish this, most vaccines introduce a weakened or inactivated version of a virus (or some “recognizable” protein from a virus) so the body can learn to detect and defeat that virus. Pfizer and Moderna made headlines in 2020 for using a groundbreaking new approach years in the making.
Rather than introducing a weakened copy of the coronavirus (or one of its recognizable proteins), their COVID-19 vaccines introduce strands of messenger RNA (mRNA). These strands act like sets of instructions that tell the body’s cells to produce copies of a recognizable (but harmless) virus protein. In this case, SARS-CoV-2’s famous “spike” protein.
Briefly flooded with harmless copies of the spike protein, the immune system learns to recognize the spikes, mounts a defense against them, and develops antibodies to guard against future infection.
Dr. Simone Blayer, global head of chemistry, manufacturing, and control at PATH’s Center for Vaccine Innovation and Access, says that how these vaccines are made is just as novel—and just as amazing—as how they work.
Faster by an order of magnitude
Making mRNA vaccines means manufacturing RNA, a very complex process that requires several highly purified ingredients. First, you must be able to manufacture DNA plasmids—the templates upon which the RNA is built.
“This step is roughly as complex as creating a whole vaccine in the first place,” Simone says with a laugh. “The good news is that DNA plasmid manufacturing is already well-established. And once you have the DNA templates, that’s when incredible speed starts knocking on your door.”
“Once you have the DNA templates, that's when incredible speed starts knocking on your door.”— Dr. Simone Blayer, Center for Vaccine Innovation and Access, PATH
To understand just how much faster this approach is, you need to understand the normal pace of viral vaccine production. To begin manufacture of a viral vaccine, you need lots of animal cells, each one infected with a weakened (or dead) virus.
For some vaccines, like the one against yellow fever, these cell cultures are grown in chicken eggs (LOTS of chicken eggs). For others, like the vaccine against meningitis A, the cells are grown in a fermenter (picture a giant, stainless steel pressure-cooker).
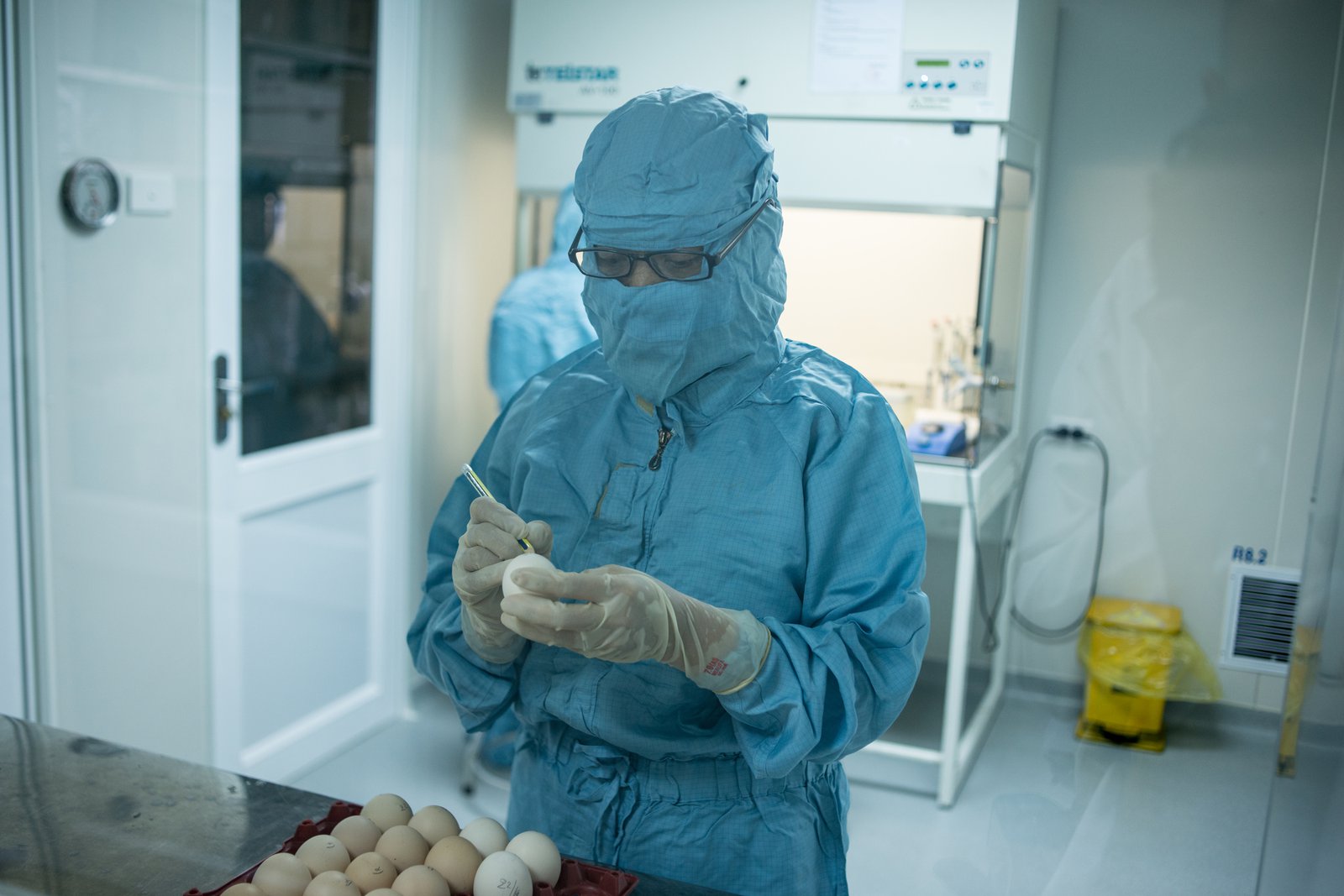
In Vietnam, a lab technician at the Institute of Vaccines and Medical Biologicals inspects eggs that will be used for vaccine manufacturing. Photo: PATH/Matthew Dakin.
Simone says that “even with modern fermentation equipment, reaching adequate biomass to begin manufacture of a viral vaccine takes about four to six weeks. Once underway, each growth and production cycle might take a week. An mRNA vaccine is synthesized in a matter of minutes.”
The incredible difference in speed is owed to the fact that viral vaccines rely on animal cell biology while RNA manufacturing is a cell-free, biochemical process performed with synthetic enzymes.
Which brings us to the other highly purified ingredients required for RNA manufacturing: chemically derived ribonucleotides. These are the little building blocks (you might know them as G, A, U, C) that RNA polymerase synthesizes to create the desired strand of mRNA.
The future of vaccine manufacturing
If RNA vaccine manufacturing is so much faster, will it replace other kinds of vaccine production?
According to Simone, that’s not likely. “The world will continue to use mRNA vaccine technology, and researchers will apply it to some new diseases as they emerge. But you shouldn’t expect it to replace existing vaccine technologies anytime soon.”
He explained several reasons why. First is that the success of a new vaccine technology is generally determined by the safety and efficacy of the vaccines, rather than by manufacturing efficiency.
Second is world’s path dependency on current knowledge and equipment. Most vaccine manufacturers (including PATH partners like the Serum Institute of India) are already set up with the expertise and equipment needed to produce biologic vaccines—from long-standing formulations for chickenpox and polio to newer adenovirus vaccines like the human papillomavirus (HPV) vaccine and Oxford–AstraZeneca’s COVID-19 vaccine (which the Serum Institute is producing).
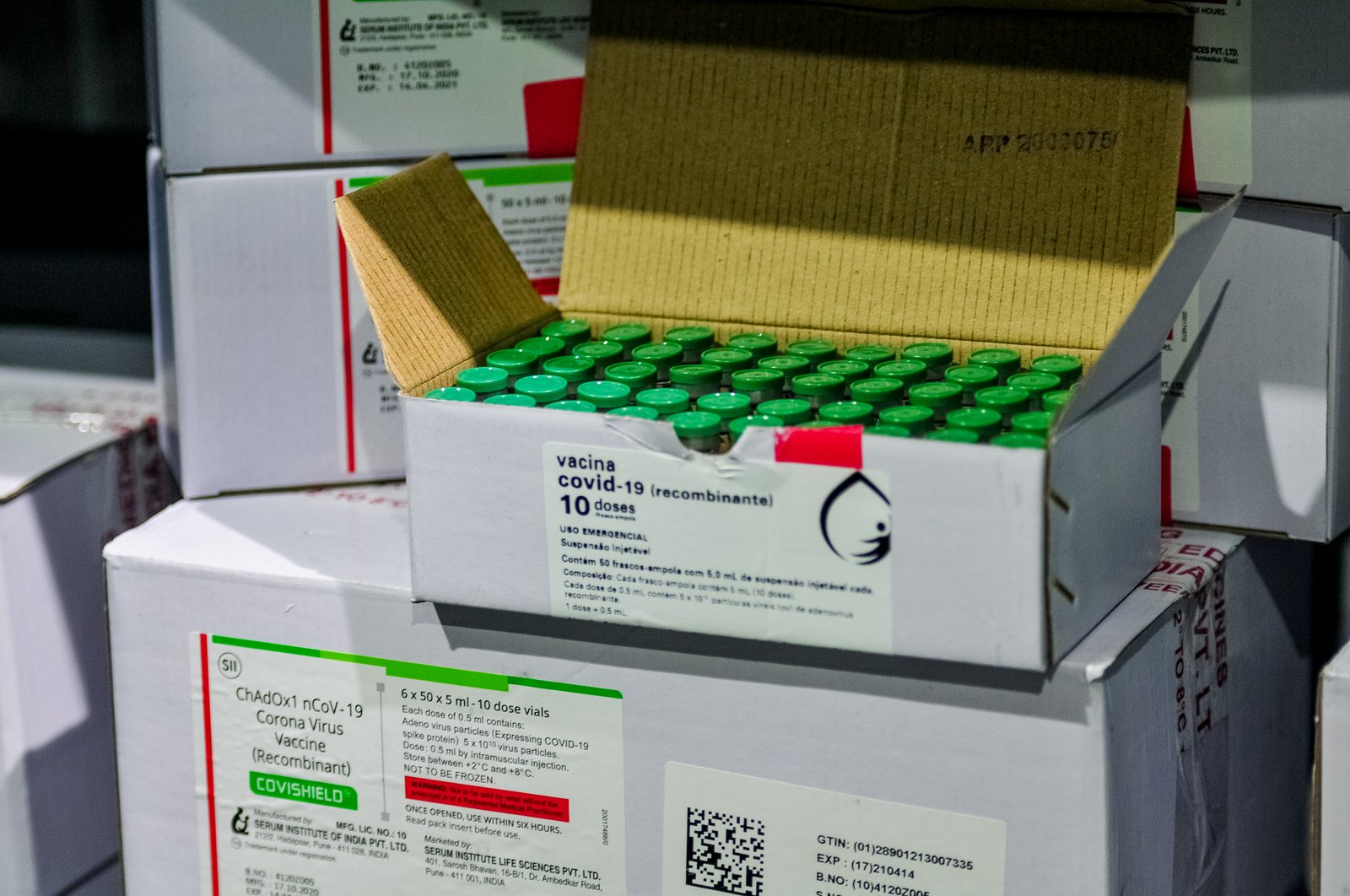
At a clinic in Brazil, a shipment of the Oxford–AstraZeneca COVID-19 vaccine produced by the Serum Institute of India. Photo: Agência Brasília/Joel Rodrigues.
Simone says that “it’s not impossible, but it’s highly unlikely that RNA, or any new vaccine technology, would be applied to a disease for which there is already a proven solution with an affordable price.”
Which brings us to the third factor: cost. RNA vaccines require new and expensive technology—not just on the manufacturing side, but on the distribution side as well. Because RNA is so fragile, all RNA vaccines would require ultra-cold chain storage, which carries enormous costs and significantly limits usability in countries with low- and middle-income economies.
“RNA vaccines are really exciting because they allow us to create a new vaccine rapidly—a big advantage for responding to outbreaks of new diseases,” Simone says. “But they are still an altogether new class of vaccines. We need a long-term track record of their use. We need more pharmacovigilance data: on efficacy in population, on side effects. So far the data are encouraging.”